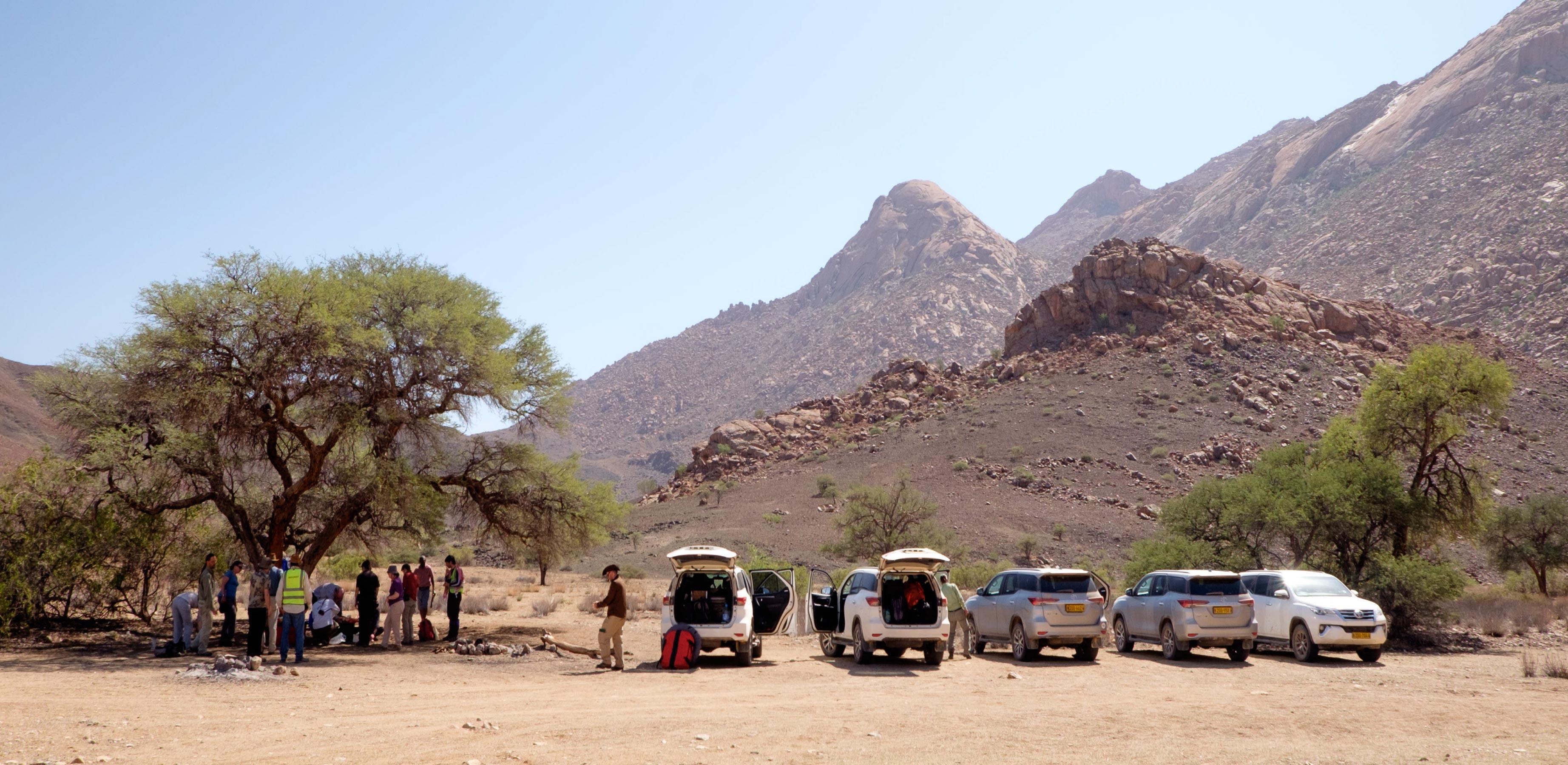
Submitted by Dr C.M. Martin-Jones on Sat, 05/08/2023 - 11:20
The move from fossil fuels toward renewables is underway. But the energy transition requires large amounts of raw materials — such as copper, nickel and lithium — for use in anything from wind turbines to car batteries. How can research at Cambridge help in locating these critical supplies? Charlie Beard, postdoctoral research associate working on the geodynamic and magmatic controls of mineralisation, explains.
The rollout of renewable energy generation and electrified transport infrastructure requires mining of a range of raw materials, many of which have historically low demand. Can a process-based understanding of the formation of these critical geological resources help us to efficiently locate them, and to extract from them with minimal environmental impact?
The biggest challenge that society faces today is, of course, climate change. Over the last 20 years use of "green" technologies, including solar, wind power, and electric vehicles, has grown massively. The good news is that this growth has driven down cost, so that renewables are now comparable with, or often cheaper than, fossil-based options. The adoption of renewable technologies is climbing sharply but globally in 2020 usage was still less than 10%. Building clean energy and transport infrastructure requires materials; a greater diversity of materials than fossil-fuel-based counterparts. Realising a low-carbon future will therefore be very mineral intensive and will require much geological expertise.
The raw materials that we need to support the energy transition include both well-established commodities such as copper, and materials that society has not mined in significant amounts before. The motors that drive EVs and the generators in wind turbines use high-strength REE-Fe-B magnets. Solar uses Ga, In, Te, and Se; minor metals that have not been produced in significant amounts in the past. Battery storage requires Li, Co, graphite, Ni, and Mn. Connecting it all, the electrical grid uses Cu, Zn, Ag and more. All are essential ingredients for the energy transition. Some are termed 'critical' because there are concerns around security of supply and where they are being mined at the moment.
Recycling will be really important and by the end of this century we may be approaching something that looks close to a circular economy. But right now almost every EV ever made is still on the road. Every wind turbine is still spinning away. There's almost nothing to recycle. And on top of that no process is 100% efficient, so we will always loose some material in manufacture and use, however hard we try. The shift in material demand is so large that an "optimistic" industry forecast is that, by 2040, we may get no more than 10% of our energy-critical materials from recycling. So we obviously need to mine primary geological resources. The good news is that many energy-critical materials have low historical demand. Because society hasn't yet looked very hard, there are likely to be many near-surface deposits to find. Geologists are now exploring in a wide range of environments and there's lots to learn!
Drill core from the Catalão II Nb-P deposit, Alto Paranaíba Igneous Province, Brazil.
In Cambridge, Owen Weller, Carrie Soderman and I are focussed on alkaline igneous rocks and their contained deposits of REE and HFSE. The REE are critical for manufacture of magnets, high-performance optics, and catalysts, and the HFSE are mostly used in high-performance alloys, abrasives, medical devices and nuclear reactor control rods. Our work examines the processes by which alkaline magmatic systems form, and seeks to quantify the processes that lead to the concentration and mineralisation of their contained metals, or to the formation of barren systems.
We use a "mineral systems" approach, tracing the path of metals from source rocks where they reside in low concentrations prior to deposit formation. A carrier for the metal, perhaps a silicate melt, hydrothermal fluid or brine is important, as is a geodynamic trigger that provides the energy kick that sets the system into motion. Once the metal is mobile, it needs a path to travel through; such as a dyke or vein system. Then the metal must be concentrated, and deposited in a trap, usually by crystallisation from a fluid or melt. Finally, the freshly-formed mineral deposit must be preserved. If erosion has washed the metals away, or sedimentation buried it beyond the depths accessible by mining then it is of no use to us. If any of these six 'critical processes' has not occurred, there is no mineral deposit. Best to move on and look elsewhere.
A process-based understanding is useful to streamline exploration, first looking at the continental-scale, then using progressively higher-resolution and more specialised geological techniques to zoom in. The ultimate aim is to streamline exploration for high‐grade deposits of critical raw materials, from which extraction can yield the greatest amount of metal for the smallest environmental impact and energy use. The easier and cheaper it is to find deposits of materials critical for renewable energy technologies, the faster society can move away from fossil fuels.
This article first appeared in GeoCam Issue 20. You can find the magazine online here.